Next-Generation Magnetic Device Generated from Extraterrestrial Material -Future-oriented manufacturing by integration of nanotechnology and planetary science (Press Release)
- Release Date
- 16 Dec, 2009
- BL25SU (Soft X-ray Spectroscopy of Solid)
- BL39XU (Magnetic Materials)
Japan Synchrotron Radiation Research Center
Hiroshima University
High Energy Accelerator Research Organization
The University of Tokyo
Japan Synchrotron Radiation Research Center (JASRI; Tetsuhisa Shirakawa, President), Hiroshima University, the High Energy Accelerator Research Organization, and The University of Tokyo have jointly discovered a new magnetic material in meteorites that can be effectively used for next-generation magnetic devices. The magnetic properties of iron meteorites greatly differ from those of iron-nickel alloys found on Earth, the reason for which has long been veiled in mystery. The scientists of this research team approached this mystery of the magnetic properties of iron meteorites by accurately evaluating their physical properties from the viewpoint of materials science and, at the same time, considering that such accurate evaluation will be effective for the exploration of other magnetic materials. They directly observed iron meteorites at the nanometer level using a photoemission electron microscope (PEEM)*1 at SPring-8, and discovered a new magnetic domain structure,*2 which has never been found in conventional iron-nickel alloys. (PEEM is a cutting-edge microscope that came under the spotlight because of its use in the research awarded the 2007 Nobel Prize in Chemistry.) By comparing the magnetic domain structure obtained in the experiments with that obtained by simulation, it was clarified that the magnetic domain structure originates from tetrataenite,*3 an iron-nickel phase unique to iron meteorites. This tetrataenite phase, originating from the universe, does not contain rare metals and exhibits excellent functionalities; therefore, it is expected to lead to the achievement of high density and power saving as well as resource saving in next-generation magnetic devices. Currently, the artificial creation of tetrataenite and the evaluation of its physical properties are ongoing, aiming towards its application to such devices, which are expected to have a productive ripple effect on future green nanotechnology. The results of this research were achieved by a joint research team consisting of the research scientist Masato Kotsugi (JASRI), Chief Scientist Chiharu Mitsumata (Hitachi Metals, Ltd.), Professor Hiroshi Maruyama (Hiroshima University), Associate Professor Kanta Ono (High Energy Accelerator Research Organization), and Professor Masaharu Oshima (The University of Tokyo). The achievements were published in the scientific journal Applied Physics Express on 18 December 2009, and also received the Japan Institute of Metals Best Micrograph Award. Publication: |
<Figure>
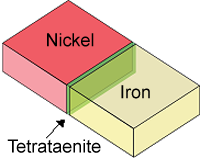
Fig. 2 Schematic of interface in Widmanstatten structure
The interface structure can be modeled as a magnetic multilayered film comprising nickel, tetrataenite, and iron.
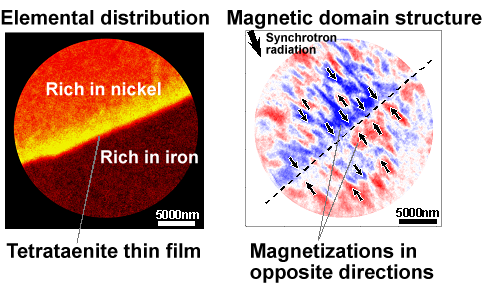
Fig. 3 Magnetic domain structure and elemental distribution in the vicinity of iron-nickel interface
Magnetization in opposite directions was observed on both sides of the interface. This is a mysterious magnetic domain structure that has never been found in conventional iron-nickel alloys.
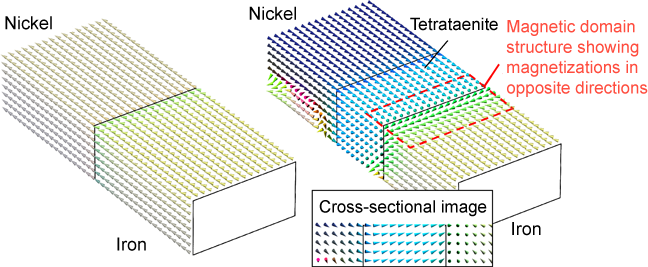
Fig. 4 Magnetic domain structure obtained by micromagnetics simulation
(a) Magnetic domain structure at simple iron-nickel interface. (b) Magnetic domain structure at iron/tetrataenite/nickel interfaces. The magnetic domain structure with magnetizations in opposite directions was simulated in the vicinity of the interface and found to originate from tetrataenite.
(a) Conventional iron/nickel interface (b) Interface of iron meteorite
<Glossary>
*1 Photoemission electron microscope (PEEM)
PEEM is an electron microscope that can realize the direct imaging of spatial information of photoelectrons emitted from the surface of a sample with 20-30 nm resolution. This microscope is capable of mapping X-ray absorption intensity because the observed photoelectron intensity is proportional to the X-ray absorption intensity. The material composition, crystal structure, and spatial distribution of a magnetic domain structure can be directly observed at the nanometer level using this principle; therefore, the use of PEEM is rapidly becoming widespread in nanotechnology.
*2 Magnetic domain structure
The magnetic domain is a ferromagnetic region where the directions of the magnetic moment are aligned.
*3 Tetrataenite
Tetrataenite is composed of 50% iron and 50% nickel, and characterized by a regular periodic structure of iron and nickel atoms that are alternately ordered. Tetrataenite is an iron-nickel phase unique to iron meteorites and has not been found on Earth. In general, laminar tetrataenite is localized at the interface between the iron-rich α–phase and nickel-rich γ–phase in the Widmanstatten structure. Here, a phase of an iron-nickel alloy with a nickel composition of 25% or lower is called an α–phase and that with a nickel composition over 25% is called a γ–phase. Tetrataenite, which is the mineralogical name, is also called γ' in metallurgy and L10-type iron nickel in crystallography.
For more information, please contact: |
- Current article
- Next-Generation Magnetic Device Generated from Extraterrestrial Material -Future-oriented manufacturing by integration of nanotechnology and planetary science (Press Release)