Clarification of Mechanism Underlying Pathogenesis of Hereditary Musculoskeletal Disorder by High-Brilliance X-Ray Diffraction Analysis - Diagnosis using small amount of biopsy sample becomes possible (Press Release)
- Release Date
- 13 May, 2010
- BL45XU (RIKEN Structural Biology I)
Japan Synchrotron Radiation Research Institute
Hiroyuki Iwamoto, a senior scientist, and Naoto Yagi, a chief scientist, of Japan Synchrotron Radiation Research Institute (JASRI; Tetsuhisa Shirakawa, President), and Drs. Julien Ochala and Lars Larsson of Uppsala University (Sweden) clarified the mechanism underlying the pathogenesis of myopathy (with symptoms such as reduced muscle power) by X-ray diffraction analysis. Myopathy is caused by the change (point mutation) in only one amino acid among the 284 amino acids constituting tropomyosin, a protein that regulates the contraction and relaxation of skeletal muscles. In this point mutation, arginine, the 133rd amino acid in tropomyosin, is replaced by triptophan (this phenomenon is called β-tropomyosin mutation (R133W)). Muscle fibers obtained by biopsy from R133W patients were examined using BL45XU RIKEN Structural Biology I, a small-angle X-ray scattering beamline, at SPring-8, and the findings were compared with those obtained from healthy subjects. It was found that the structural change of tropomyosin molecules, which are required for the activation of skeletal muscles, was inhibited in the R133W patients. This study was performed at SPring-8 with the approval of JASRI as a Medical Bio EX Proposal. This study demonstrated that X-ray diffraction analysis is a revolutionary diagnostic method that enables clarification of the cause of genetic diseases using a small biopsy sample. The clarification of the mechanism underlying the pathogenesis of myopathy due to R133W will greatly contribute to the development of therapeutic medicines. These achievements were published online in the American academic journal, Proceedings of the National Academy of Sciences of the United States of America, on 10 May 2010. Publication: |
<Figure>
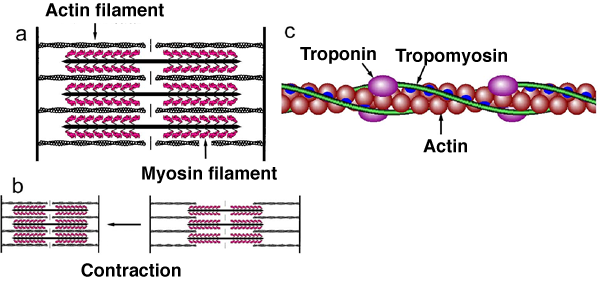
a. Structure of one sarcomere (the basic unit of myofibril for contraction). There are two types of filament; actin and myosin filaments, both of which are involved in muscle contraction.
b. Muscle contraction occurs when the actin and myosin filaments slide together and overlap.
c. An enlarged image of an actin filament, showing the positional relationship among contraction regulatory proteins, troponin, tropomyosin, and actin molecules.
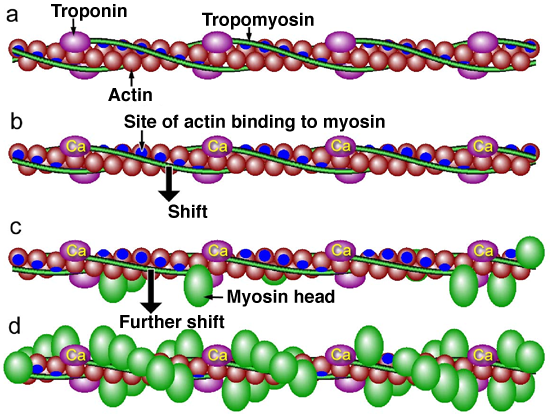
a. Without calcium ions (in relaxed state). Because tropomyosin completely covers the sites (indicated in blue) of actin binding to myosin, myosin cannot bind to actin.
b. When calcium ions released from excited muscle cells bind to troponin, the binding sites of actin are exposed owing to the shift of tropomyosin; however, the extent of the shift is not sufficient for myosin to bind to actin.
c. When small amounts of myosin heads bind to the binding sites of actin under state b, tropomyosin shifts further, resulting in the complete exposure of the binding sites of actin.
d. Because the binding sites of actin are completely exposed, many myosin heads can bind to actin, which enables the generation of a large muscle contraction power.
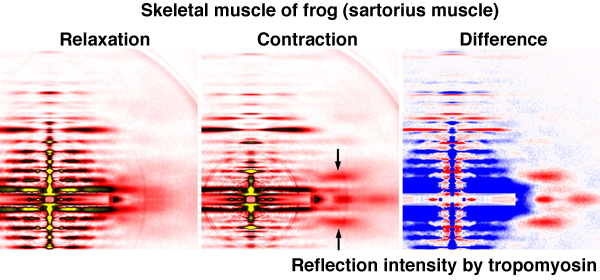
The left, center, and right images show the patterns when the muscle is relaxed, contracted, and the difference between the two, respectively. Muscle contraction was induced by electric stimulation. In the right image, the areas in which X-ray diffraction intensity increases and decreases during muscle contraction are indicated in red and blue, respectively. The reflection intensity by tropomyosin (arrow) increases during contraction, indicating the shift of tropomyosin, as shown in Figs. 2(b) and 2(c).
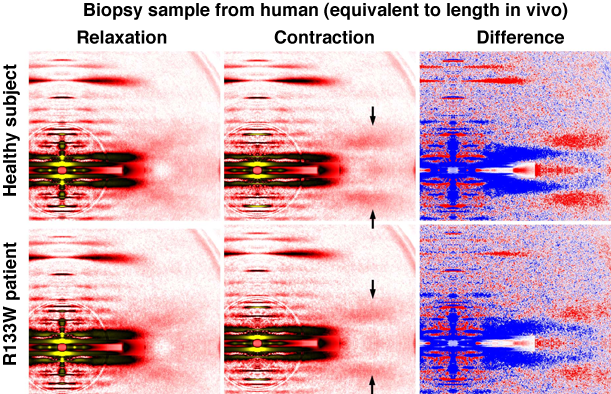
The patterns were recorded when actin and myosin filaments sufficiently overlap (Fig. 1(b), left). The contraction of the muscle was induced by adding calcium ions. The reflection intensity by tropomyosin (indicated by arrow) is intensified during muscle contraction in the healthy subject, similarly to skeletal muscle contraction in the frog. On the other hand, the increase in the reflection intensity by tropomyosin is small in the R133W patient.
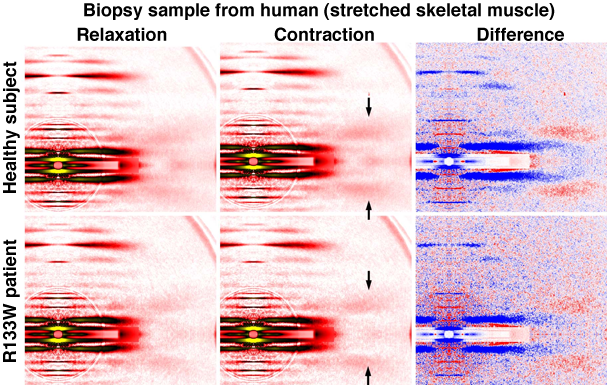
The patterns were recorded under stretched condition so that there is no overlap between actin and myosin filaments (Fig. 1(b), right). In the healthy subject, the increase in the reflection intensity by tropomyosin is smaller than that shown in Fig. 4 because no muscle activation due to myosin occurs; however, the increase is much smaller in the R133W patient. This finding indicates that the shift of tropomyosin caused by the presence of calcium ions (Fig. 2(b)) is inhibited in the R133W patient.
For more information, please contact: |
- Current article
- Clarification of Mechanism Underlying Pathogenesis of Hereditary Musculoskeletal Disorder by High-Brilliance X-Ray Diffraction Analysis - Diagnosis using small amount of biopsy sample becomes possible (Press Release)