Real-Time Monitoring of Ultrahigh-Speed Initial Processes of Photochemical Reaction Using Free Electron Laser (FEL) - Observing the Moment with Two Ultrashort Pulsed Lights: FEL and Femtosecond Laser (Press Release)
- Release Date
- 01 Apr, 2010
- SCSS
RIKEN
Japan Synchrotron Radiation Research Institute
Key research findings
• Observation of photochemical reaction by time-resolved photoelectron imaging using synchronized ultrashort pulsed light
• Real-time monitoring of rapid changes in electron state reflecting the reaction state in a molecule
• Verification of practicality and effectiveness of research on ultrahigh-speed phenomena using superlight called X-ray FEL (XFEL)
RIKEN (Ryoji Noyori, President) and Japan Synchrotron Radiation Research Institute (JASRI; Tetsuhisa Shirakawa, President) have succeeded in monitoring the initial processes of photochemical reactions in organic molecules in real time by synchronizing a free electron laser (FEL)*1 and a femtosecond (fs; a femtosecond is a quadrillionth of a second) laser.*2 This was achieved by Suet Yi Liu, an Asia Program associate, Yoshihiro Ogi, a cooperative scientist, and Toshinori Suzuki, a chief scientist at Chemical Dynamics Laboratory, all of RIKEN Advanced Science Institute (Kohei Tamao, Director), and by a research group under the SPring-8 Joint Project for XFEL (Nobuo Fujishima, Director) jointly organized by RIKEN and JASRI. Scientists of the SPring-8 Joint Project for XFEL are working to achieve oscillation of the XFEL,*3 which is designated as a national critical technology. XFEL is a totally new light that combines the features of synchrotron radiation (intense X-ray) and laser (coherent high-quality light), and is considered to be a superlight. XFEL may enable the monitoring of various ultrahigh-speed phenomena at the atomic and molecular levels because of its high brilliance and very short pulse duration. Hence, there is keen competition in the research and development of XFEL among European countries, the US, and Japan. In particular, XFEL is expected to enable new experiments when synchronized with a femtosecond laser; for example, one pulsed light is irradiated to a material and the ultrahigh-speed change that occurs immediately after the irradiation is analyzed by irradiating another pulsed light. This research group synchronized FEL with wavelength in the vacuum ultraviolet region, a small prototype XFEL (SCSS test accelerator),*4 with a femtosecond laser with wavelength in the ultraviolet region, and successfully monitored, for the first time, the initial processes of photochemical reactions occurring in organic molecules on a picosecond (ps; a picosecond is a trillionth of a second) time scale by time-resolved photoelectron imaging.*5 The monitoring experiment was carried out by connecting the photoelectron imaging setup developed by the research group at RIKEN Advanced Science Institute to the SCSS beamline. First, the ultraviolet femtosecond laser was irradiated onto and absorbed by pyrazine,*6 an aromatic chemical, and then FEL was irradiated onto pyrazine, after a delay, to release electrons from molecules. By measuring the distribution of these emitted electrons, the ultrahigh-speed initial processes of photochemical reactions called internal conversion and intersystem crossing*7 were clearly observed. The experiment in this research was the first laser synchronization experiment using the FEL constructed by RIKEN, and also the world's first experiment in studies on photochemical reactions using a wavelength-variable ultraviolet femtosecond laser and a vacuum ultraviolet FEL. In the future, this will be a marked step towards research on chemical reactions using XFEL. The results of this research will soon be published as a rapid communication in the scientific journal of the American Physical Society, Physical Review A. Publication: |
<Figure>
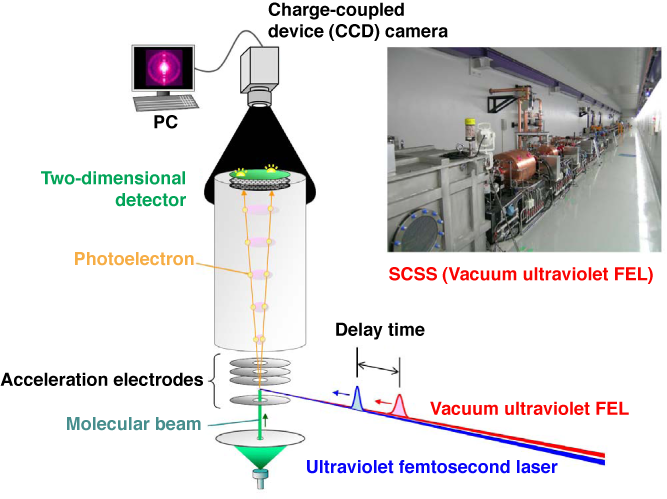
Fig. 1 Schematic of experimental setup for time-resolved photoelectron imaging using synchronized vacuum ultraviolet FEL and ultraviolet femtosecond laser, and photograph of SCSS test accelerator
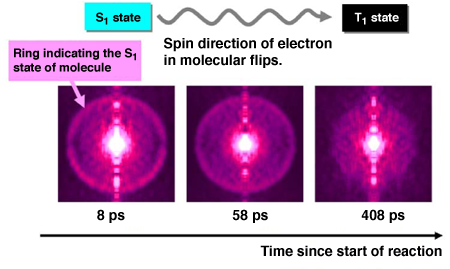
Fig. 2 Image of photoelectrons illustrating electron state of molecule during reaction
The result of an experiment using 324-nm-diameter ultraviolet femtosecond laser as the first optical pulse and 161-nm-diameter FEL as the second optical pulse is shown. The photoelectron images with three different delay times (time since the start of the reaction due to the irradiation of the first optical pulse) are shown in order of delay time. The ring indicating the S1 state of the molecule gradually disappears with increasing delay time.
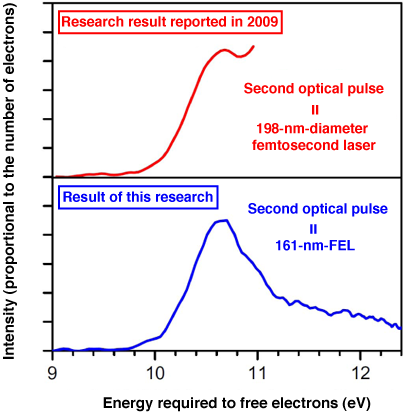
Fig. 3 Photoelectron spectra obtained by analyzing photoelectron images
The result of an experiment using 260-nm-diameter ultraviolet femtosecond laser as the first optical pulse and 161-nm-diameter FEL as the second optical pulse is shown. In the research result reported in 2009, a 198-nm-diameter ultraviolet femtosecond laser was used as the second optical pulse and the spectrum ended at about 11 eV. In this research, the entire spectrum was clarified using the 161-nm-diameter FEL as the second optical pulse.
<Glossary>
*1 Free electron laser (FEL)
When (free) electrons are accelerated to near-light velocity and weave between periodically positioned magnets, light is emitted from the electrons at every change of direction and interacts with the electron beam. The synchronized light gradually becomes coherent (wave peaks and troughs are respectively alighted) and amplified, and oscillates as FEL.
*2 Laser synchronization
Optical pulse oscillation is timed by transmitting pulse signals with a predetermined reference frequency to multiple laser devices.
*3 X-ray free electron laser (XFEL)
XFEL is a FEL with a wavelength in the X-ray region. It is designated as a national critical technology as it exhibits the world's highest performance as a research and development technology that can lead to other Japanese scientific technologies. The construction of XFEL facilities started in FY2006 and is scheduled to be completed in 2010. Similar projects are also ongoing in the US, where successful laser oscillation was achieved, and the European countries (e.g., Germany); thus, there is tough competition among Japan, the US, and European countries.
*4 SCSS test accelerator
The SCSS test accelerator is a prototype XFEL. SCSS is the abbreviation for SPring-8 Compact SASE Source, where SASE stands for self-amplified spontaneous emission and is a method of oscillating a laser by amplifying light without using reflection mirrors. For details, see the press release dated 22 June 2006, "Successful lasing of the X-ray Free Electron Laser (XFEL) prototype."
*5 Time-resolved photoelectron imaging
After a reaction is induced or molecular motion is controlled by the first optical pulse, the molecules are ionized by the second optical pulse to allow them to emit electrons (photoelectrons). The three-dimensional distribution (data on velocity and angle) of these emitted photoelectrons is recorded as images by time-resolved photoelectron imaging.
*6 Pyrazine
Pyrazine is an aromatic compound with a planar six-membered ring structure and is represented by C4H4N2. In photochemistry, pyrazine is considered to be a typical molecule that undergoes the intramolecular reactions called internal conversion and intersystem crossing*7 upon the absorption of ultraviolet radiation.
*7 Internal conversion and intersystem crossing
In the process where the electron energy of excited molecules is converted to vibration energy and the electron state changes, there are two cases: no electron spin (rotation) changes and one electron spin flips. The former is called internal conversion and the latter is called intersystem crossing.
For more information, please contact: Dr. Yoshihiro OGI |
- Previous Article
- Clarifying New Phase of Water - One Step Closer to Clarification of Mysterious Properties of Water (Press Release)
- Current article
- Real-Time Monitoring of Ultrahigh-Speed Initial Processes of Photochemical Reaction Using Free Electron Laser (FEL) - Observing the Moment with Two Ultrashort Pulsed Lights: FEL and Femtosecond Laser (Press Release)