Topic 20: Developing Ultra-High Resolution Spectroscopy based on Nuclear Resonance
Developing Amazing New Spectroscopic Technologies in the Quest to Determine the True Nature of Materials
The functions and combinations of the constituent elements determine the properties of a material; herein the functions and properties of each element are determined by the states of the constituent atoms and electrons. Mössbauer spectroscopy is an indispensable technique to fully understand the states of atoms and electrons, which are the fundamental components of a material. However, the application of Mössbauer spectroscopy in high-energy regions has been difficult due to limitations arising from the measurement mechanisms. To overcome this difficulty, Dr. Makoto Seto (Professor, Kyoto University, Japan) and colleagues developed new spectroscopic techniques applicable to high-energy regions by utilizing highly brilliant synchrotron radiation at SPring-8. Through their efforts, precise spectroscopic analyses for numerous elements, including almost all rare earth elements, are now possible.
Limitations of Mössbauer Spectroscopy in Examining Atoms and Electrons
Mössbauer spectroscopy is a technique to examine states, such as valences, electronic structures, and magnetic properties, of elements in a material. It utilizes a phenomenon where nuclei of elements are resonantly excited by absorbing the gamma (γ)-ray energy, which is irradiated with γ-rays with a specific energy. Thus, the radioactive isotopes of target elements are the main γ-ray sources. In particular, Mössbauer spectroscopy using γ-rays emitted from iron (Fe) has been extensively utilized not only in physics and chemistry, but also in biology and earth science. In 2004, samples collected by the Mars probe were analyzed using Mössbauer spectroscopy, revealing that water was once present on Mars. Despite these successes, with the exception of a few elements such as iron, preparing a radioisotope radiation source suitable to measure each element had been difficult. Moreover, analyzing elements that can only be measured using a very short-lived radioisotope had been nearly impossible.
“Although several ideas, which utilized high-intensity synchrotron radiation, had been proposed to overcome these difficulties, other issues remained. For example, new high-speed, high-precision detectors, which can tolerate high-intensity synchrotron radiation, needed to be developed for measurements for Mössbauer spectroscopy in high-energy regions (> 30 keV). Hence, many hurdles remained before realizing measurements of nuclei requiring high excitation energy,” explains Dr. Seto.
Hyperfine interactions are interactions between electron systems and nucleus systems. These interactions are utilized to measure the structures and magnetic properties of electrons around the nuclei of a specific atom using the resonant excitation phenomena. This technique provides information about the structures and magnetic properties of such electrons without disturbing the electron systems by precisely measuring the transition of a nuclear energy level and removing degeneracy. The transition of a nuclear energy level (a quantum mechanical view of the discrete magnitude relation of energy) from one level to another is induced by the effects of the structures and magnetic properties of such electrons. In contrast, degeneracy (a quantum mechanical term describing two or more different physical states at the same energy level) is removed by splitting the degenerate energy levels.
Developing Ultra-High Precision Spectrometry Required Perceptual Changes
Traditionally, Mössbauer spectroscopy using synchrotron radiation utilizes nuclear resonant forward scattering. This technique measures quantum beats, or more specifically the variations in the intensity of scattering γ-rays in the forward direction induced by mutual interference among many different energy levels of nuclei, when the nuclei are resonantly excited by pulsed synchrotron radiation. However, it is difficult to use high-energy synchrotron radiation in this technique. When high-energy γ-rays are emitted or absorbed, the nuclei recoil, reducing the energy of γ-rays, decreasing the likelihood of the Mössbauer effect. Thus, Dr. Seto and colleagues shifted their thinking, and realized Mössbauer spectroscopic measurements using high-energy synchrotron radiation.
They aimed to accomplish high-precision spectrometry by detecting the internal conversion electrons and fluorescent X-rays. When an excited state nucleus returns to a lower energy level, the internal conversion occurs where orbital electrons, instead of γ-rays, are emitted. The emitted electrons are called internal conversion electrons. Irradiating with X-rays whose energies exceed an element-specific value excites the inner-shell electrons (electron in the inner orbits) of the constituent atoms of a target material and the outer-shell electrons (electron in the outer orbits) transit into the holes created by the excitation. This element-specific energy is called the absorption edge energy. Because the energy levels of outer-shell electrons are higher than those of inner-shell electrons, X-rays with energy equal to this energy difference will be emitted upon this transition. These X-rays are called fluorescent X-rays.
Achieving Success in Mössbauer Spectroscopy using Highly Brilliant Synchrotron Radiation
Although spectral data can be obtained from internal conversion electrons and fluorescent X-rays, this is insufficient for Mössbauer spectroscopy using synchrotron radiation due to the low measurement precision and efficiency. To overcome these issues, Dr. Seto and colleagues developed a technique to obtain data about the properties of a sample by concurrently using a reference sample with known properties. Then they measured the differences in atomic energies between these two samples (Fig. 1 and explanation therein). This technique provides sufficient precision to measure nuclei with high-energy levels. Their research group conducted an experiment using a well-documented sample of Fe oxide, hematite (α-Fe2O3), at SPring-8's JAEA Quantum Dynamics Beamline (BL11XU) to determine if Mössbauer spectroscopy can be performed using this technique. Their demonstration experiment revealed that the resultant spectra agree with known spectra obtained using radioactive isotope sources (Fig. 2). Moreover, they conducted an additional experiment to measure the absorption spectra of an isotope of germanium (Ge), specifically the 68.752 keV third excited state of 73Ge, which has high-energy, short-lived excitation levels, using the Nuclear Resonant Scattering Beamline (BL09XU). Then they successfully applied Mössbauer spectroscopy using synchrotron radiation to measure the absorption spectra of 73Ge, which was difficult to obtain because suitable radioactive isotope sources were unavailable (Fig. 3). Their results clearly demonstrated that Mössbauer spectroscopic analyses are possible on numerous elements, including almost all rare earth elements.
“Small sample measurements, imaging measurements, and measurements under complex extreme environmental conditions were very difficult until the development of Mössbauer spectroscopy using synchrotron radiation, but now they are easy. This new technique is also applicable to energy regions that are covered by existing techniques, which allows complicated spectral analyses to be conducted utilizing the vast amounts of existing data. Furthermore, spectrometry with a resolution at the nanoelectron volt level (neV = 10-9 eV) has become possible for nuclear excitations with very narrow energy widths (~1 neV) and quasi-elastic scattering (scattering energy is moving and scattering varies with time),” explains Dr. Seto.
Their research achievements were published in Physical Review Letters (May 29, 2009). Moreover, this method should promote research on the Earth's internal structure.
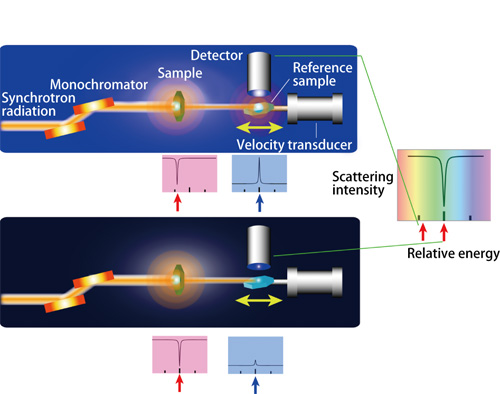
Synchrotron radiation X-rays are initially irradiated to a sample and the transmitted X-rays are sequentially irradiated to a reference sample. Fluorescent X-rays emitted from the reference sample are detected when nuclear resonant excitations occur. To measure absorption spectra, the scattering intensity of fluorescent X-rays is measured by varying the energy of the incident X-rays to a reference sample. To vary this energy, the Doppler effect, which is induced when the reference sample is moved by a velocity transducer, is utilized. When the energy of the fluorescent X-rays emitted from a sample (a dip in a top red plot) differs from that of the reference sample (top), synchrotron radiation X-rays with energy that induces nuclear resonant excitations in the reference sample (a bump in a top blue plot) will not be absorbed in the sample. This results in nuclear resonant excitations in the reference sample (the left-hand side red arrow in a right-most plot). However, when these two energies are equal (bottom), synchrotron radiation with energy to induce nuclear resonant excitations in a reference sample will be absorbed by the sample (a dip in a bottom red plot). Thus, nuclear resonant excitations will barely occur in the reference sample (a small bump in a bottom blue plot). Therefore, precise data of the scattering intensity reveals that absorption dips when the resonant excitation energies are equal (a dip indicated by a right-hand side arrow in a right-most plot). Conversely, if the locations of such absorption dips are identified, then the energy states of an unknown sample can be determined.
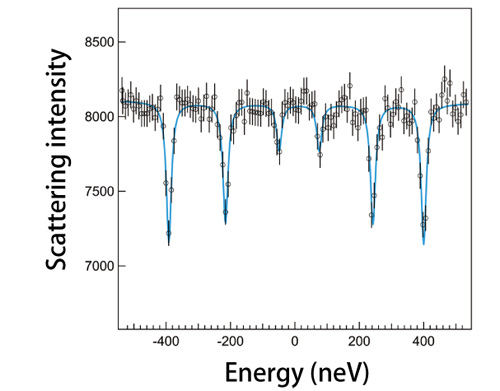
Sample and references sample are iron oxide (α-Fe2O3) and palladium (Pd) metal containing 2% Fe atoms, respectively
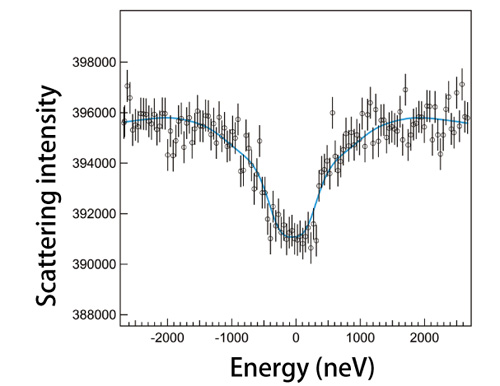
Sample and reference sample are lithium germanate (Li2GeO3) and a germanium oxide (GeO2), respectively
Reference
1. M. Seto, R. Masuda, S. Higashitaniguchi, S. Kitao, Y. Kobayashi, C. Inaba, T. Mitsui and Y. Yoda; Phys. Rev. Lett., 102, 217602 (2009)