Water Molecules Still Shrouded in Mystery - Unlocking the secrets of the mysterious structure and patterns of motion of water molecules
Reasons for still studying the structure of water
Water is very familiar to us and its simple molecular structure, H2O, is well known even to junior high school students. Although it is hard to believe that the state of water has not yet been completely clarified, water is still shrouded in mystery. Although people tend to think that scientific research only targets unknown and novel phenomena, it is an important task of scientific research to attempt to find and clarify mysterious phenomena in common substances such as water and ice. You may be surprised to hear that the structure of water is being studied at SPring-8, one of the world's leading optical scientific research facilities. However, there are good reasons for carrying out such research. Have you ever wondered where ice stores its enormous amount of cold energy (energy for cooling objects), why the density of water is greatest at 4°C and decreases both below and above 4°C, or why the density of solid ice is lower than that of liquid water? To answer these questions, it is essential to clarify the structure of water, an issue that is still generating controversy. Recently, the structural analysis of water and ice has been carried out at SPring-8, and some new facts that may lead to the resolution of some long-standing arguments about the nature of water have been revealed.
Hydrogen bonding between water molecules
There are six electrons in the outermost orbital of oxygen atoms and one electron in the orbital of hydrogen atoms (Fig. 1). When an oxygen atom and two hydrogen atoms bond to form a water (H2O) molecule, the hydrogen atoms share their single electron with the orbital of the oxygen atom but also share an electron with the oxygen atom, thus forming a strong bond. Hydrogen and oxygen atoms are stabilized when their outermost orbitals are filled with two and eight electrons, respectively. Therefore, strong bonds called covalent bonds are formed between the two hydrogen atoms and the oxygen atom. Thus, hydrogen and oxygen atoms form a stable structure by sharing their electrons.
Individual H2O molecules are considered to exist independently and stably, so why do they form solid crystals (ice) or a liquid with a maximum density at 4°C (water)? The key to unveiling this mystery is believed to be the hydrogen bond, a mutual attraction mechanism between H2O molecules. Because the electrons in the two hydrogen atoms are attracted to the oxygen atom by the covalent bond, the side of the hydrogen atom opposite the covalent bond is slightly positively charged, whereas the remaining four electrons in the outermost orbital of the oxygen atoms, which are not used in the covalent bonds, form two lone pairs and are negatively charged (Fig. 2). Therefore, the two positively charged sides of the hydrogen atoms in an H2O molecule attract the negatively charged lone pairs in two other H2O molecules, whereas the two negatively charged lone pairs attract the positively charged sides of the hydrogen atoms in two other H2O molecules. The thus-formed bonds are called hydrogen bonds (Fig. 3), and their bonding strength is estimated to be approximately one-tenth that of covalent bonds.
The above mechanism means that a H2O molecule can attract four other H2O molecules around itself. It is easy to imagine five H2O molecules placed at the four vertices and center of a regular tetrahedron. Such base structures are regularly arranged to form crystals, i.e., ice. Traditionally, the bonding state between H2O molecules in ice and their functions were difficult to directly observe and were therefore analyzed by molecular dynamics simulations, namely, by simulating the mutual interaction between virtual atoms and its mechanism using a computer. Recently, however, scientists have succeeded in directly observing the structure and functions of ice in a high-precision Compton scattering experiment using the high-energy inelastic scattering beamline (BL08W) at SPring-8, verifying the accuracy of molecular dynamics simulations.
Compton scattering is used to examine the patterns of motion of electrons in target molecules and atoms by measuring the difference in photon energy before and after X-ray particles (photons) collide with electrons and then scatter. In the above study, it was clarified that part of the cold energy in ice is stored in a network structure, in which the bonding strength of H2O molecules increases with decreasing temperature, and that the remaining energy is stored as the vibration energy of H2O molecules. This fundamental research is expected to contribute to the development of heat-storage materials and to clarifying the heat-storage characteristics of new materials in the future, and was published in a top US scientific journal.
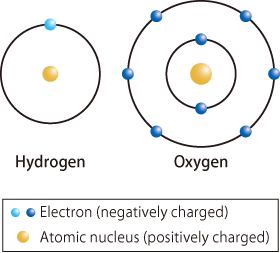
fig1.Schematic of hydrogen and oxygen atoms
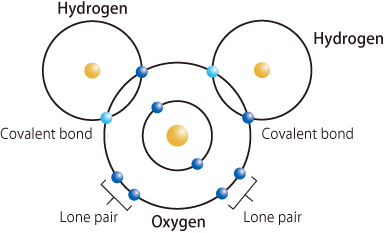
fig2.Schematic of H2O molecule and covalent bonds
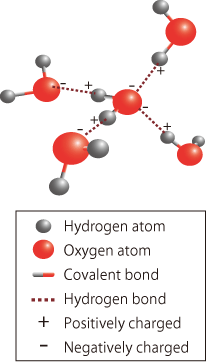
fig3.Schematic of four hydrogen bonds of H2O molecule
Hydrogen atoms covalently bonded to highly electronegative atoms, such as oxygen and nitrogen atoms, are slightly positively charged because the electrons of hydrogen atoms are attracted to the electronegative atoms. This enables the formation of weak bonds with lone pairs in adjacent water molecules with a bonding force approximately one-tenth that of the covalent bonds. These bonds are called hydrogen bonds. In the case of H2O molecules, two out of the six valence electrons in oxygen atoms are involved in the two O-H bonds, and the remaining four valence electrons form two lone pairs, thus enabling the formation of a total of four hydrogen bonds with adjacent water molecules.
The mysterious structure of water
In 1892, Röntgen, who discovered X-rays and won the first Nobel Prize in Physics, proposed a model for water in which an ice-like structure and an unknown structure are mixed to form water. In 1933, however, after investigating X-ray diffraction data for water, professors at Cambridge University proposed a theoretical model for water in which the structure of ice, comprising H2O molecules placed at the four vertices and center of a regular tetrahedron, is continuously distorted to form water. This model was not universally accepted but was supported by many scientists because corroborative results were obtained in various subsequent spectroscopic analyses and three-dimensional molecular dynamics simulations carried out using computers, the performance of which has been rapidly improving since the 1980s.
In 2008, Takashi Tokushima, a research scientist of RIKEN, and his colleagues analyzed the structure of water using high-brilliance soft X-rays from the RIKEN Physical Science III Beamline (BL17SU) and a soft X-ray emission spectrometer with the world's highest resolution equipped with a newly developed special container (a liquid flow cell) with windows made from strong 150-nm-thick thin films with a high transmittance for soft X-rays. When an electron is expelled from the inner orbital of an oxygen atom covalently bonded to hydrogen atoms upon the irradiation of soft X-rays, an electron in the outer orbital moves to the inner orbital to occupy the positively charged vacant position (a hole). At this time, the energy corresponding to the difference between the energy of the electron in the inner and outer orbitals is released as soft X-rays. Therefore, we can determine the state of lone pairs by examining differences in the energy distribution of the released soft X-rays (energy spectrum). This is the principle of the structural analysis of water by soft X-ray emission spectroscopy (Fig. 4). When the soft X-ray emission spectrum of water (liquid) is examined by this method, two peak intensities corresponding to the lone pairs involved in hydrogen bonds are observed (Fig. 5). This finding suggests that two states of water with different patterns of hydrogen bonds simultaneously exist. To examine this finding more precisely, the scientists analyzed the structure of water using various techniques, for example, small-angle X-ray scattering analysis, a method of observing fine structures by analyzing the scattering pattern of X-rays that are scattered with a small angle upon the irradiation of X-rays onto a material, and X-ray Raman scattering to observe molecular orbitals without electrons.
The above analyses revealed a highly unexpected structure for water. Unlike the conventionally accepted model in which the orderly ice structure is continuously and gradually distorted to form the uniform structure of water, water appears to have a relatively large variation in density (dense/thin), namely, ice-like orderly structures (with a low density) are sparsely distributed in a high-density “sea” of H2O molecules that are distorted due to the breaking of hydrogen bonds, resulting in polka-dot-like fine structure (Fig. 5). It was also found that the variation in density becomes small at high temperatures and large at low temperatures. Because of this finding, it is necessary to reappraise the model proposed by Röntgen. Since the results were published in an American scientific journal, there have been calls to modify the new model of water based on molecular dynamics simulations, and a lively debate about the nature of water is still ongoing. The density of ice being lower than that of water and the density of water being greatest at 4°C now appear to be partially explainable by considering the intermolecular distance and the average number of H2O molecules surrounding a H2O molecule (the arrangement number). However, there are still many unclear mechanisms, for example, the rate of change between the two states of water, and how this rate of change is affected when a substance is dissolved in water. Thus, the mysteries of water have not yet been fully clarified.
You may be surprised to hear that the above-mentioned fundamental research on water is indispensable in clarifying the mechanism underlying the dissolution of substances in water and the roles of water in biological organisms and chemical reactions. However, for example, to clarify the functions of biological cells containing water with various ions, it is necessary to fully understand the structure of water and the states of the relevant electrons. Thus, the expectation that SPring-8 will lead the world in fundamental research on water is growing.
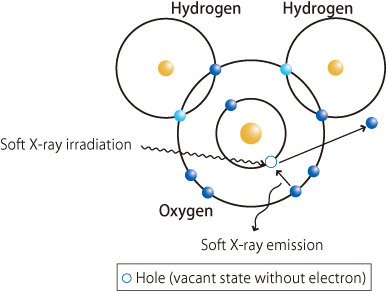
fig4.Schematic of soft X-ray emission spectroscopy
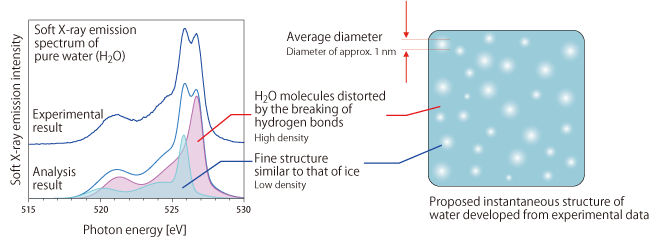
fig5.Proposed structure of water developed from previous experimental data of soft X-ray emission, small-angle X-ray scattering, and X-ray Raman scattering
(Left) Two curves obtained in a new experiment, i.e., one indicating a group of H2O molecules distorted by the breaking of hydrogen bonds and the other indicating a fine structure similar to that of ice. These curves correspond to a single curve suggesting two different states of water, previously obtained by analyzing the emission spectrum of water (H2O) at room temperature.
(Right) The proposed structure of water. The three-dimensional structure is expressed in a two-dimensional plane for simplicity. When the water temperature increases, the density of high-density components decreases and approaches that of low-density components and the polka-dot contrast representing the density becomes small. Therefore, it becomes difficult to observe the difference between these components by small-angle X-ray scattering. The above structure is constantly changing with the recombination of hydrogen bonds (which recombine in 1-2 picoseconds; a picosecond is one-trillionth of a second). Therefore, changes in the water structure can be continuously detected if we use methods for obtaining information with femtosecond resolution (a femtosecond is one-quadrillionth of a second), i.e., faster than the recombination of hydrogen bonds, such as soft X-ray emission, small-angle X-ray scattering, and X-ray Raman scattering.
Interview and original text by Narichika Honda