Topic 22: Deciphering the Chemical Compositions of Mantle Transition Zone
Experimentally Measuring the Seismic Velocity in the Deep Mantle
Earth, whose radius is ~6,400 km, is covered by a thin crust. Beneath this crust is a 2,900 km thick lithosphere, called the mantle. The mantle has two seismic discontinuities at depths of 410 and 660 km where the seismic wave velocities and densities suddenly increase. The debate about the principal mineral of the mantle transition zone sandwiched by these two seismic discontinuities remains controversial. To resolve this dispute, high-pressure, high-temperature conditions equivalent to the transition zone must be reproduced. Then the velocities of these experimentally produced seismic waves (elastic waves) must be compared to actual seismic waves (elastic waves) measured on the ground. Recently researchers have successfully reproduced conditions equivalent to Earth's deep interior using a large-scale high-pressure generator at SPring-8, and they identified a principal mineral in the transition zone as well as obtained valuable clues about the lower transition zone.
Debate—Olivine or Carbuncle?
The mantle, which occupies 83% of the Earth's volume, is divided into the upper mantle, mantle transition zone, and lower mantle by two seismic discontinuities (Fig. 3). Although the principal minerals of the upper mantle are magnesium/iron silicate minerals, also called olivine, which is a sort of gem commonly known as peridot, there are various models for these minerals in the mantle transition zone. The two most prevalent models are: (1) olivine is the principal mineral of the mantle transition zone and (2) carbuncle is the principal mineral of the mantle transition zone. Carbuncle is a mineral composed of various elements, including calcium, magnesium, and iron, and is a sort of gem known as garnet. A special type of carbuncle created under high pressure is called majorite. The debate over these two models has raged for the last 20 years without reaching a consensus.
Seismic waves such as P-waves and S-waves, which can be observed on the ground, are clues about the conditions in Earth's interior. Depth-dependent propagation velocities of seismic waves have been determined with a high degree of accuracy. Therefore, by assuming candidate minerals in Earth's interior based on the data obtained from seismic waves and measuring seismic velocities (elastic wave velocities) of these candidates, the best candidate minerals can be determined. Because the mantle transition zone is at a pressure of 20 GPa (GPa = 109 Pa = ~10,000 atm), ground experiments are difficult. Thus, the best candidate, olivine or carbuncle, remained unclear until recently.
Revealing the Principal Mineral in the Mantle Transition Zone
In April 2005, Dr. Tetsuo Irifune (Professor, the Geodynamics Research Center, Ehime University, Japan), Dr. Yuji Higo1) (Researcher, ditto), Dr. Toru Inoue (Associate Professor, ditto), Dr. Yoshio Kono (Researcher, ditto), Dr. Hiroaki Ofuji (Assistant Professor, ditto), Dr. Ken-ichi Funakoshi (Associate Senior Scientist, JASRI), and colleagues initiated research to end the debate.
It is important to experimentally reproduce the conditions equivalent to the mantle transition zone on the ground. Examples of high-pressure phases where the materials with identical compositions exhibit different properties under high-pressure conditions, such as carbon becomes diamond at ~5 GPa, are commonly reported. The high-pressure phase of olivine is ringwoodite, while that of carbuncle is majorite. Dr. Irifune and colleagues succeeded in in situ X-ray measurements of minerals at pressures exceeding 20 GPa using the High Temperature and High Pressure Research Beamline (BL04B1) at SPring-8. They published their initial research results in Science (1998).
To examine materials in the mantle, the seismic velocities should also be measured under high-pressure, high-temperature conditions. Although the State University of New York (SUNY), USA is a global leader in measurement techniques of such seismic velocities, their highest measurement pressure was limited to ~10 GPa. Thus, Dr. Irifune and colleagues combined their pressure generating techniques with SUNY's techniques and realized measurements under higher pressure conditions. Dr. Irifune explains the experimental procedure, “We irradiated ultrasounds possessing the same properties as a seismic wave onto ~2 mm sample, and measured the transit time of the ultrasounds through the sample. Additionally, we precisely measured the sample length upon irradiation with ultra-highly brilliant X-rays at SPring-8” (Fig. 1).
Their research group was the first to successfully determine the seismic wave velocities in ringwoodite and majorite under conditions at up to ~20 GPa and 1,400 °C, which are equivalent to those in the mantle transition zone (Fig. 2). Comparing their experimental results to seismologically obtained velocity data revealed that the principal mineral of the mantle transition zone is olivine. Thus, the carbuncle models were rejected.
However, one question, which even the olivine models could not explain, remained about the properties of seismic waves from the lower part of the mantle transition zone. Thus, their research group further examined the data obtained at SPring-8, and found that harzburgite, which is a form of olivine known as orthopyroxene peridotite, is the best mineral to explain the seismic velocities in the lower part of the transition zone immediately above the 660 km deep seismic discontinuities.
1) Currently Research Scientist at Japan Synchrotron Radiation Research Institute (JASRI).
Are “Plate Graveyards” Possible?
Understanding that harzburgite is the principal mineral in the lower part of the mantle transition zone immediately above the 660 km seismic discontinuity has important implications that go beyond identifying the principal mineral. This knowledge suggests “plate graveyards” exists.
Harzburgite is the principal material of subducted plates, and stagnant slabs exist near subducted plates at a depth of ~660 km (e.g., directly under the Japanese islands). These stagnant slabs are thought to sink down into the lower mantle when they reach a certain size. By combining this understanding with the recent understanding that harzburgite is the principal mineral of the lower part of the transition zone, it is possible that stagnant slabs exist in the form of layers from a few tens of km to ~100 km depths across the entire globe. This is the area where subducted plates spread, and is called “plate graveyards” (Fig. 3).
Several models have been proposed to explain plate movements. Similar to flushing a toilet, in flushing models, plates sink down in the lower mantle and induce cataclysmic crustal movements when plates reach a depth of 660 km. In contrast, the giant hot plume models postulate a synchronized action where hot materials, which correspond to the mass of sinking cold plates, move up from the boundary between the mantle and core as the plates sinks. However, if plate graveyards broadly exist, many plates should remain in the ~600 km region. In other words, unlike the Japanese popular novel Nippon Chinbotsu (Japan Sinks), cataclysmic crustal movements due to the sudden sinking of plates would not occur. Their research achievements were published in Nature (February 2008), and received international attention.
Currently, their research group is developing new technologies to measure seismic velocities under conditions exceeding 25 GPa to explore materials in even deeper parts of the lower mantle as well as to measure the seismic velocities of oceanic crustal materials, which are other types of constituent materials of the plates. Moreover, they plan to conduct further research to confirm the existence of plate graveyards and to explore the possibility of flushing. Accumulation of data from these measurements will gradually, but steadily, elucidate the structures and dynamics of Earth's deep interior as well as Earth's evolution and creation processes.
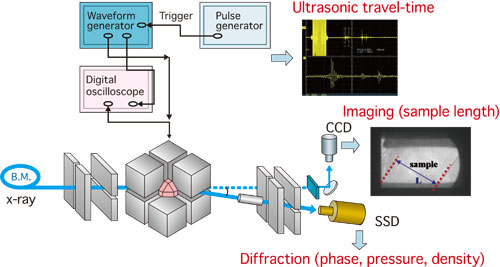
Propagation velocities of seismic waves (elastic waves) were measured using small samples that were pressurized and heated. Variations in sample lengths were also determined by simultaneously irradiating ultrasounds onto the samples.
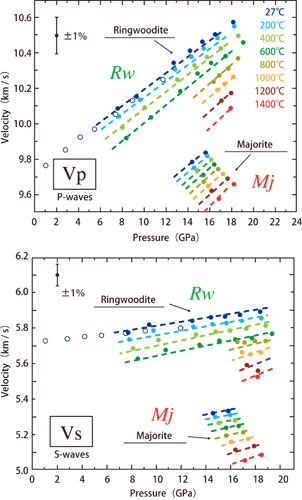
Elastic wave velocities of both minerals increase with pressure or temperature. However, majorite has smaller velocities than ringwoodite.
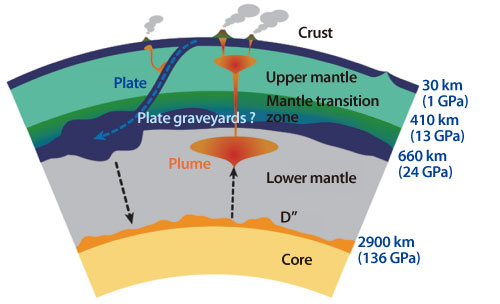
This research revealed that similar to the upper mantle, the mantle transition zone is mainly comprised of olivine. Additionally, it suggests the existence of “plate graveyards” under the mantle transition zone.
References
1. T. Irifune, N. Nishiyama, K. Kuroda, T. Inoue, M. Isshiki, W. Utsumi, K. Funakoshi, S. Urakawa, T. Uchida, T. Katsura and O. Ohtaka; Science, 279, 1698 (1998)
2. T. Irifune, Y. Higo, T. Inoue, Y. Kono, H. Ohfuji and K. Funakoshi; Nature, 451, 814 (2008)