Topic 23: Synthesizing Minerals of Uranus and Neptune
Discovering the Principal Minerals Hidden within the Cores of Ice Giants
Uranus and Neptune are called ice giants because their major constituent is ice. However, the cores of these ice giants are composed of rocks and metals, and their compositions were once believed to be similar to the solid part of the Earth. Unfortunately, only the average density of the entire planet can be observed, and the core's condition in an ice giant is unknown. However, the realization of ultra-high pressure conditions of 300 GPa (GPa = 109 Pa = ~10,000 atm) at the High Pressure Research Beamline (BL10XU) at SPring-8 has uncovered another candidate for the core of an ice giant, silicon dioxide (SiO2). The ultra-high pressure conditions have revealed structural variations in SiO2, leading to the discovery that a new mineral may exist deep within ice giants.
Examining Phase Transitions of Silica at a Pressure of 300 GPa
Terrestrial planets such as Mercury, Venus, and Mars have cores composed of metals such as iron and are surrounded by a mantle. The mantle, which is comprised of various minerals, is covered by a thin layer of rock, called the crust. In contrast, the mantle of ice giants such as Uranus and Neptune are composed of ice, whose main element is water. However, ice giants have cores composed of solid elements, which are similar to the mantle and crust of the terrestrial planets, but the core of an ice giant is believed to be much denser than the solid elements in terrestrial planets, including Earth.
The mantles and crusts of the terrestrial planets and the cores of Jovian planets (gas-giant planets) are composed of various minerals, whereas the principal mineral in the cores of ice giants is speculated to be silicon oxide (SiO2). Silicon is the 7th most abundant element in the universe. Thus, SiO2 is an important component of the solar system. SiO2 is generally called silica and is in a crystalline form, termed quartz1), under normal conditions.
However, placing silica under high-pressure, high-temperature conditions changes its structural state (phase) but not its composition (SiO2). A structural change without altering the composition is called a phase transition. A good example of the phase transition is freezing water to make ice or pressuring graphite, which consists of carbon, at 5 GPa converts it to diamond.
The High Pressure Beamline (BL10XU) at SPring-8, which provides the world's highest pressure and temperature conditions, was further upgraded in Spring 2005. The maximum pressure was increased from 220 GPa at 2,200 °C to 300 GPa at 2,000 °C. Dr. Kei Hirose2) (Associate Professor, Tokyo Institute of Technology (Tokyo Tech), Japan) and colleagues at the Japan Synchrotron Radiation Research Institute began experiments to study the phase transitions of silica under the high-pressure, high-temperature conditions available at the upgraded BL10XU.
1) Colorless and transparent quartz is typically called quartz crystal, which is valuable as a gem.
2) Currently Professor at Tokyo Institute of Technology and is affiliated with the Institute for Research on Earth Evolution (IFREE), Japan Agency for Marine-Earth Science and Technology.
Extracting Information from Small Sample Quantities using Highly Brilliant X-rays
Prior to their experiments at the upgraded BL10XU, a research group at Tokyo Tech and IFREE led by Dr. Hirose discovered in 2004 that MgSiO3 post-perovskite is a principal mineral of the D” (D double-prime) layer, which is a boundary region (depth of 2,700-2,900 km) between the lower mantle and outer core (see Topic 21 for more details). This achievement was realized using the extraordinarily high pressure (125 GPa), high temperature (>2,200 °C) conditions only available at BL10XU beamline of SPring-8. “Elucidation of the phase transition of silicon, which is an abundant mineral in the universe and solar system, would be an invaluable clue in exploring the deep structures of planets other than the Earth, especially ice giants,” Dr. Hirose explains the aim of their observation experiments on the phase transition of silica under the extraordinary high-pressure, high-temperature conditions.
To further pursue these studies, a laser-heated diamond anvil cell high-pressure generator was developed (Fig. 1). A crystalline silica sample was held in the gap between two diamonds placed face-to-face at their tips. Then this configuration was compressed by squeezing these diamonds while heating with laser irradiation. This system realized a pressure of 300 GPa and 2,000 °C. However, this powerful system has a drawback: the sample size is limited. To realize such extraordinary high-pressure conditions that exceed 200 GPa, the current limit of the gap between these two diamonds is 10 μm (μm = 10-6 m) and the sample must be less than half that of the gap. The scattering and diffraction intensities of X-rays irradiated onto such a small sample are very weak, which significantly limits the information that can be obtained. Fortunately, the capabilities of SPring-8 can overcome this drawback. “The highly brilliant X-rays obtained at BL10XU of SPring-8, which are 108 times brighter than those obtained from existing conventional X-ray sources, finally allowed us to obtain useful data,” says Dr. Hirose.
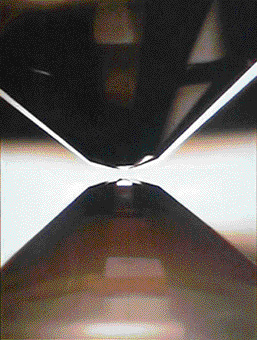
Crystalline silica sample is held in a gap between two brilliant-cut diamonds, which is subsequently compressed by squeezing these two diamonds and heating with an irradiating laser. Gap is only 5 μm.
Is Silica a Major Element in the Cores of Uranus and Neptune?
Dr. Hirose and colleagues measured the X-ray diffraction of pressurized silica. When the applied pressure exceeded 270 GPa, the hexagonal cylinder-shaped crystal structure changed into a dice-shaped structure. “We concluded this is a new pyrite-type mineral,” explains Dr. Hirose. This new mineral is called a pyrite-type because it resembles a golden, dice-shaped pyrite crystal composed of iron disulfide (FeS2). Thus, Dr. Hirose and colleagues successfully converted silica into an unknown form under the ultra-high pressure, ultra-high temperature conditions available at SPring-8.
More interestingly, this new mineral is much denser than other known silica minerals. In fact, the existence of pyrite-type silica was theoretically predicted in the 1980s by several research groups, including some Japanese groups, but it was not discovered until the ultra-high pressure conditions were realized at SPring-8. Pyrite-type silica, which is more than twice as dense as a quartz crystal at ordinary temperature and pressure, was predicted to be a metal, but is not. A pressure level of 270 GPa corresponds to the outer core of the Earth. Because iron is the major component of Earth's outer core, this new mineral does not naturally exist on Earth. Their research achievement was published in Science (August 2005), and received worldwide attention from planetary scientists.
“However, the cores of ice giants such as Uranus and Neptune may be composed of this pyrite-type silica,” speculates Dr. Hirose. This research provides an important clue in solving the mystery of ice giants because actual measurements cannot be performed using existing technologies.
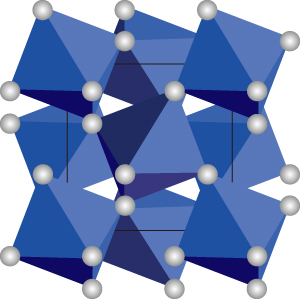
Fig. 2. Crystal structure of pyrite-type silicon dioxide (SiO2).
White circles represent oxygen. Silicon atoms are situated at the centers of octahedrons indicated by the blue color.
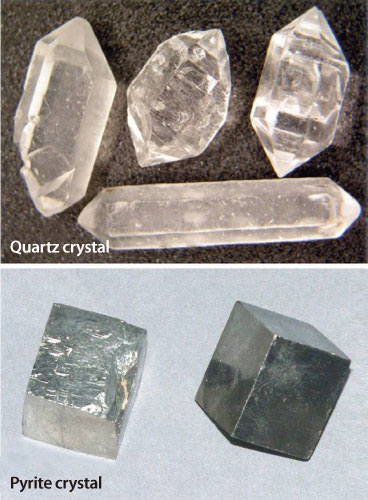
Fig. 3. Quartz crystal and pyrite crystal.
Quartz crystals are hexagonal cylinder-shaped, whereas pyrite crystals are dice-shaped.
Reference
1. Y. Kuwayama, K. Hirose, N. Sata, Y. Ohishi; Science, 309, 923 (2005)