Topic 10: Developing Quasicrystals Comprised of Three-Component Block Copolymers
“Quasicrystals” - A Mysterious Material Discovered in Polymers
Quasicrystals, which have similar structures but different properties than crystals, have drastically changed the framework of crystallography. Until a Japanese research team discovered quasicrystals in polymers, quasicrystals had only been observed in alloys. Polymeric quasicrystals are 50-100 nm (1 nm = 10-9 m), which are enormous (more than 100 times larger) than those found in alloys. Compared to common materials, quasicrystals refract light in the opposite direction (negative refraction index). Due to this property, quasicrystals should contribute to the development of a “superlens,” which will focus on images of objects smaller than the light wavelength. Moreover, because polymeric materials are easily designed, quasicrystals have great potential in practical applications. Although polymeric quasicrystals are relatively large, the domain size of quasicrystals is about 5 μm (1 μm = 10-6 m). Therefore, SPring-8 is indispensable for structural analyses.
Discovering Quasicrystals in Polymers
In 1982, materials scientist Dr. Dan Shechtman (Professor, the National Institute of Standards and Technology1), USA) discovered an unfamiliar state that differed from common crystals in aluminum-manganese alloys where primitive unit cells (basic building blocks of a crystal) were regularly aligned to comprise crystals. Diffraction spots are observed due to the interference of X-rays according to the conformation of the primitive unit cells upon X-ray irradiation of a crystal. However, the state that Dr. Shechtman discovered did not contain primitive unit cells, but some level of regularity provided the diffraction spots. Moreover, its structure was a regular icosahedron (polyhedron with 20 identical equilateral triangular faces), which is impossible for a crystal. Although it had some level of regularity (or quasi-periodicity), this material lacked the same level of regularity as the crystal. It could not be crystallographically defined but had some level of regularity (or quasi-periodicity), which was later named “quasicrystals.”
Following this original discovery, it was revealed that quasicrystals have peculiar properties, and new types of quasicrystals were found. Eventually 10-nm quasicrystals, which are much larger than the 0.5-nm size (atomic level) that Dr. Shechtman discovered, were observed. Then in April 2007 Dr. Yushu Matsushita (Professor, Nagoya University, Japan), Dr. Atsushi Takano (Associate Professor, ditto), Ken-ichi Hayashida2) (graduate student, ditto), Dr. Tomonari Dotera3) (Associate Professor, Kyoto University, Japan), and colleagues discovered for the first example of quasicrystals in complex polymers. The precise polymer-synthesizing technologies that their research group developed contributed to this achievement.
1) Formnerly the National Bureau of Standards.
2) Currently a researcher at Toyota Central R&D Labs., Inc., Japan.
3) Currently a professor at Kinki University, Japan.
Exploring the Potential of Quasicrystals using Highly Brilliant X-rays
A macromolecule is a large molecule, which is composed of mainly carbon and hydrogen and is connected by sharing electrons via covalent chemical bonds. A monomer is the basic building block of a macromolecule, e.g., the monomer of polyethylene is ethylene. The process of connecting monomers is called polymerization, and thus a macromolecule is often called a polymer. When multiple types of monomers are polymerized, the resulting polymers are called copolymers. Copolymers have several variations. A block copolymer is a common copolymer composed of chains of multiple types of monomers; each monomer chain consists of a single type of monomer consecutively connected. For example, two types of monomers A and B form a combination of monomer chains like “-A-A-A-A-A-A-B-B-B-B-B-B-.”
Block copolymers are the targets of Dr. Matsushita and colleagues. When two types of monomers (A and B) comprise a block copolymer, it is called a two-component block copolymer, which consists of an A-phase (chains of monomer A) and a B-phase (chains of monomer B). As shown in Fig. 1, the phase structure can be divided into four groups according to the length ratios of A and B. These phases range in size from a few nanometers to several tens of nanometers. Thus, this separation is called nanoscale phase separation.
On the other hand, increasing the number of monomer types to three opens a new horizon. The different components are phase-separated from each other and spatial binding forces increase to easily form a cylinder structure (Fig. 2c). Moreover, the cross-section of each cylinder forms a regularly aligned regular polygon (Fig. 2d). There are only 12 ways to fill a plane using regular polygons that are the same size, including triangles, squares, hexagons, and dodecagons (polygon with 12 sides and 12 angles). This was originally studied by a mathematician Archimedes in the 3rd century BC and was later completed by astronomer Johannes Kepler in the 17th century. For example, when the only component is a regular hexagon, (6.6.6) tiling where three regular hexagons align to their vertices is the solution. If conditions permit, polymers will spontaneously conform such a regular structure, which is called self-assembly.
Prior to starting this research, Dr. Matsushita and colleagues had already experimentally observed a block copolymer undergoing a molecular construction where three polymer components were connected via single point. This copolymer easily conformed to a cylindrical phase-separation structure. “We decided to utilize SPring-8 to examine the tiling formation of three-component block copolymers,” explains Dr. Matsushita. Their research group utilized a microbeam small-angle X-ray scattering instrument installed at the High Flux Beamline BL40XU. When irradiated, the electrons in a material scatter the X-rays. Hence, scattering with a small scattering angle can provide information about nanosized structures. Herein, X-rays at SPring-8 with an ultra-high brilliance and extremely small beam size are indispensable to explore such a tiny region.
The ratios of the three components were varied in the experiments. The polymers altered their conformations according to the components, and exhibited a series of tiling structures. Dr. Matsushita and colleagues focused on (3.3.4.3.4) tiling composed of regular triangles and squares. According to Dr. Matsushita, “We observed 12 distinctive diffraction spots of X-rays, which have not been observed in other polymers. Prior studies with other materials have revealed that such diffraction spots suggest the emergence of quasicrystals nearby.” Upon observing these unique diffraction spots, they realized that this could be the first example of a quasicrystal in a polymer.
Although they continued varying the composition of (3.3.4.3.4), quasicrystals had yet to materialize. “There was logically no question about our experimental strategy. However, we could not find them. We repeated our experiments through trial and error by encouraging staff members,” recalls Dr. Matsushita, and six month later, they finally identified quasicrystal structures (Fig. 3).
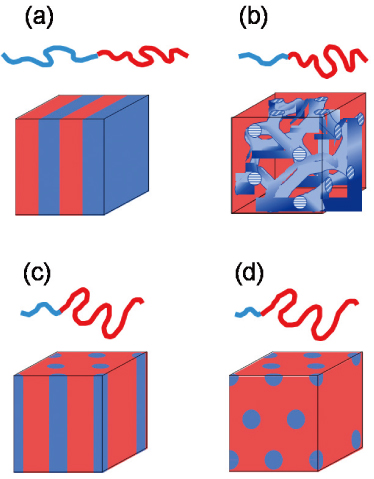
a Lamella structure. b Double gyroid (bicontinuous) structure. c Cylindrical dispersion structure. d Spherical dispersion structure.
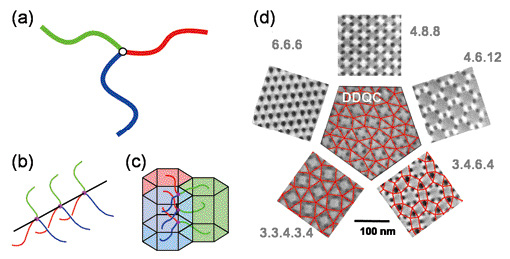
a Any combination between two green, red, or blue block chains is repulsive when they are packed in a small area due to their negative relationships. b Consequently, such repulsion in a, the points of union form a line (the black line is to guide the eye and does not exist). c Self-assembly into cylindrical aggregation structures. Points of union exist only on a line formed by gathering three hexagonal cylinders. d Cross-sections of tiling structures. Outer five structures represent Archimedean tilings, while the center represents 12-fold symmetrical quasicrystal tilings.
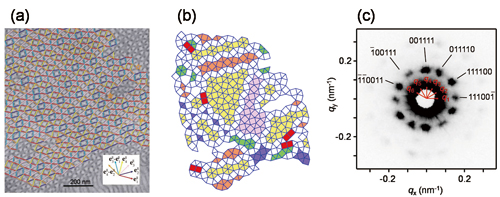
Relative fractions of three block chains comprising the sample are 1:2.7:2.5. a Wide-field transmission electron microscopic image. Virtual quasicrystal tilings are distinguished by six colors with differentiating directions. b Transition pattern diagram that is transferred from a by a regular triangle and square. c 12 diffraction spots of the 12-fold symmetrical quasicrystals obtained at SPring-8.
Possibility of Freely Designing Metamaterials
The most significant achievement of Dr. Matsushita and colleagues is that they demonstrated the universality of quasicrystals, which are commonly observed across materials with various spatial scales. Additionally, quasicrystals have potential as metamaterials. Metamaterials refract light in the opposite direction (negative refraction index) compared to common materials. When light passes through a metamaterial, a phenomenon, which can be described as the apparent bending of light around obstacles, will make previously visible materials invisible. The tile sizes of metamaterials range between 50-100 nm, and are about ten-fold larger than those in conventional materials. “If a tile size compatible with visible light is achieved by contriving molecular design methods, then it may be possible to develop a superlens with which images of objects smaller than light wavelength can be obtained. Then the development of synthetic fibers, or “transparent mantles” that could make a person wearing them invisible would not be a dream,” mentions Dr. Matsushita.
Although very few studies have investigated 100-nm (mesoscale) size tilings, the possibility of discovering various quasicrystals has been suggested. Therefore, this discovery is significant and has received both national and international attention. In particular, it has been referenced in various publications, including Nature: Research Highlights (May 2007), Physical Review Focus (May 2007), and Science: Editor's Choice (June 2007).
Reference
1. K. Hayashida, T. Dotera, A. Takano and Y. Matsushita; Phys. Rev. Lett., 98, 195502 (2007)